Carbohydrate-Based Adjuvants
Protein subunit vaccines typically require lipidation or an adjuvant for optimal function and only a handful are currently approved for human use.[1] There are no approved adjuvants for enhancing the oral, nasal or mucosal delivery of vaccines.
Protein subunit vaccines typically require lipidation or an adjuvant for optimal function and only a handful are currently approved for human use. [1] There are no approved adjuvants for enhancing the oral, nasal or mucosal delivery of vaccines.
​
Carbohydrate-based adjuvants are generally considered safe and well-tolerated and work by interacting with immune cells and signaling pathways to promote a more robust and sustained immune response. They help in the activation of antigen-presenting cells (such as dendritic cells) and stimulate the production of antibodies (specifically IgG antibodies).
​
Linear carbohydrate polymers have proven to be particularly effective as adjuvants including glycosaminoglycans, chitins, and chitosans. The best described example is hyaluronic acid (HyA), [2,3] the simplest member of the glycosaminoglycans superfamily. HyA is a natural, biocompatible adjuvant when attached to immunogenic proteins. [2-5]
​
HyA is safe, with over 200 FDA approved uses in humans. HyA is widely used in OTC cosmetics, as topical medical creams, dermal fillers, orthopedic injections, and as ingested nutraceuticals. None of these applications are vaccine related.
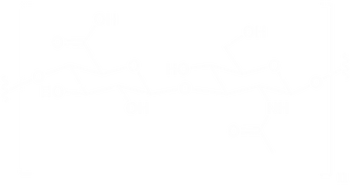
The biological activity of HyA is modulated by the size of the polymer. HyA fragments as small as 1.6 kDa elicit inflammation-related responses but play no structural role in mammalian systems. [6-8] High molecular weight HyA > 500 MDa plays a major structural role in a variety of tissues and the extracellular matrix without eliciting any immunogenic or inflammatory response. [6-8] We have identified a size of HyA, when paired with our patented covalent attachment chemistry, that effectively works as an adjuvant with most protein subunit vaccines without eliciting a deleterious inflammation response.
The underlying physiology of HyA-targeted immune receptors has been well-described. [2, 9-14] HyA binds to immune cells via multiple membrane receptors, including CD44, LYVE-1, and RHAMM while also activating toll-like receptors. HyA is believed to play an adhesive and co-stimulatory role in immunogen presenting cell activation of B and T cells [15] through these receptors and subsequent trafficking to germinal centers in the lymph nodes. [16-19]
HyA is also a potent activator of macrophages and inducer of TH1 cellular response, including high levels of IL-12 expression. This results in increased numbers of CD80 expressing cells,[20] leading to longer term immune responses and improved immune memory.
When covalently attached to protein immunogens, HyA further enhances immunogenicity of a protein vaccine. The high affinity of HyA for these immune system receptors makes it an ideal addition to lower-stimulatory immunogens for enhancement of trafficking in the lymphatic system and enhancement of immune responses to immunogen delivery.

Historically, studies using unattached HyA as an adjuvant have met with mixed success. Using our patented chemistry, HyA attachment safely occurs only at the reducing end of the carbohydrate polymer. This results in the outward presentation of the HyA polymer for improved engagement of CD44, LYVE-1 and RHAMM receptors on antigen presenting cells, and ultimately greater adjuvanting activity with improved durability of the immune response. HyA attachment DOES NOT interfere with development of an immunogenic response. Furthermore, our attachment chemistry has successfully placed HyA on dozens of different proteins and enzymes without compromising activity.
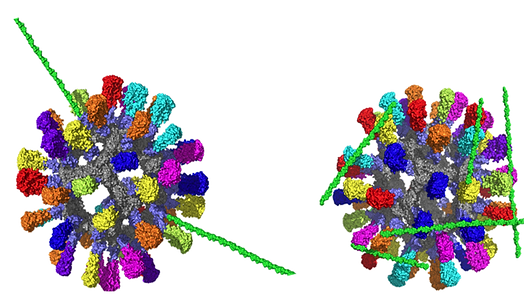
HyA (green) attachment. (Left) In vitro glycosylation of HyA on an immunogen (Right) cross-linked placement of HyA on an immunogen. The in vitro glycosylation of HyA allows the nanoparticle to better interact with the immune cell receptors.
References
1. Ramon, G. (1925)
2. Dalla Pieta, A., et al. (2021) 10.1038/s41423-021-00667-y
3. Kim, K.S., et al. (2016) 10.1002/adfm.201504879
4. Petillo, P.A. (2022) US Patent Application 63/382,020
5. Petillo, P.A., et al. (2022) US Patent Application 63/365,432
6. Gupta, R.C., et al. (2019) 10.3389/fvets.2019.00192
7. Highley, C.B., et al. (2016) 10.1016/j.copbio.2016.02.008
8. Kogan, G., et al. (2007) 10.1007/s10529-006-9219-z
9. Qi, H. (2016) 10.1038/nri.2016.94
10. Yin, X., et al. (2021) 10.1146/annurev-immunol-101819-025146
11. Durai, V., et al. (2016) 10.1016/j.immuni.2016.10.010
12. Krishnaswamy, J.K., et al. (2018) 10.3389/fimmu.2018.02169
13. Lin, C.A., et al. (2021) 10.3390/pharmaceutics13101569
14. Mallakpour, S., et al. (2021) 10.1016/j.ijbiomac.2021.05.155
15. Do, Y., et al. (2004) 10.1097/00002371-200401000-00001
16. Banerji, S., et al. (2007) 10.1038/nsmb1201
17. Campo, G.M., et al. (2010) 10.1016/j.bcp.2010.04.024
18. Jiang, H., et al. (2002) 10.1074/jbc.M108654200
19. Lesley, J., et al. (2000) 10.1074/jbc.M002527200
20. Rayahin, J.E., et al. (2015) 10.1021/acsbiomaterials.5b00181